News & Stories
Read the latest news and stories in the SickKids newsroom. Looking to interview someone? Connect with our media team.
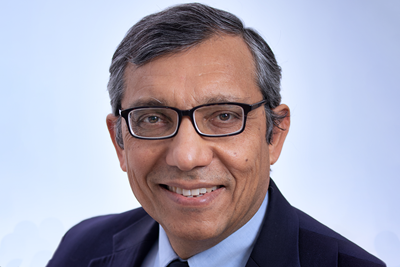
July 14, 2025
Dr. Zulfiqar Bhutta awarded 2025 Virchow Prize for global child health leadership
Bhutta has been recognized for his leadership in advancing maternal, newborn and child health equity.
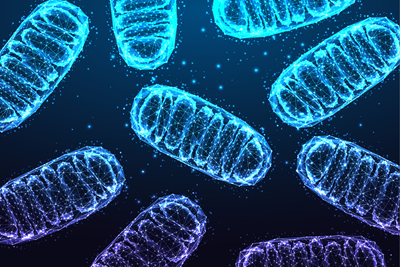
July 10, 2025
Researchers discover new way cells protect themselves from damage
International effort reveals how cell compartments form a connected defense system to maintain cellular health.
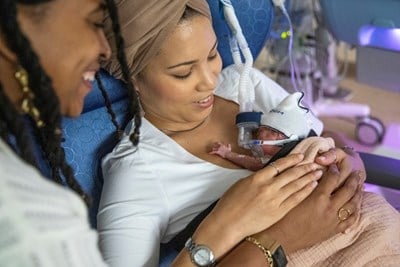
July 8, 2025
NICU families get a jump on healing with skin-to-skin “kangaroo” care
Why early skin-to-skin contact is a win-win for caregivers and their babies
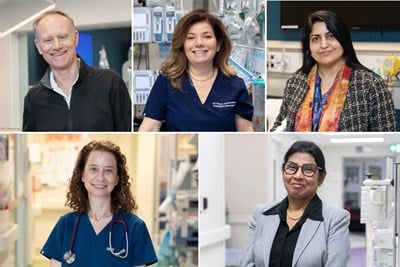
July 3, 2025
Five physicians putting their own stamp on paediatric emergency medicine education
SickKids Emergency Department is a global leader in medical education, with physicians training the next generation while delivering world-class clinical care.

July 3, 2025
The Family Centre and Children's Library has reopened
A fresh chapter begins at the Family Centre and Children’s Library.
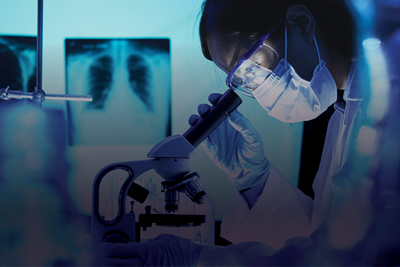
June 30, 2025
When medical research funding goes south, look to the north
After 150 years of leading Canadian medical science, our most important moment is now.
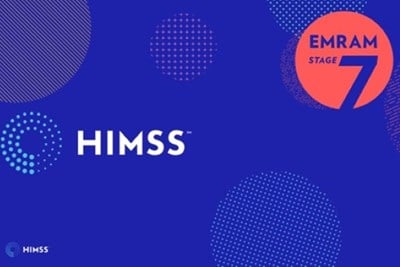
June 24, 2025
Originally awarded this prestigious designation in 2022, SickKids has now completed its first revalidation.
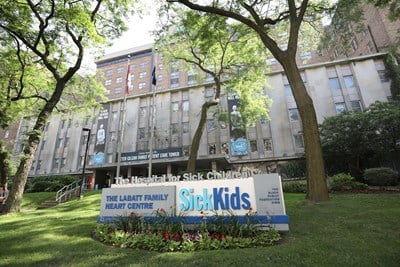
June 23, 2025
The Hospital for Sick Children (SickKids) and The Healthcare of Ontario Pension Plan (HOOPP) are pleased to announce that SickKids employees will become members of HOOPP’s defined benefit pension plan, effective Dec. 29, 2025.

June 20, 2025
New partnership project aims to bring customizable treatments to patients with genetic conditions
This project is funded by the Precision Child Health Partnership Translational Program, part of the collaborative efforts between two of Canada’s largest child health research hospitals, SickKids and CHU Sainte-Justine. The project is powered by the catalytic philanthropy of the Azrieli Foundation and their landmark grant to both institutions in December 2023.

June 19, 2025
Transforming care through research: SickKids launches Clinical Research Strategy
SickKids has launched its first enterprise-wide Clinical Research Strategy to embed research into care, driving discovery and better outcomes for patients and families.
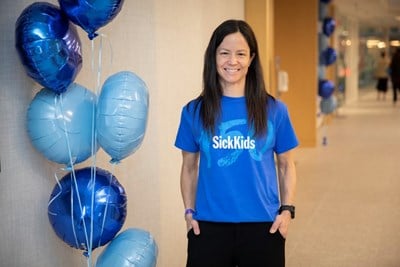
June 18, 2025
Krista Cardamone’s daughter Leah was diagnosed with cancer at just four weeks old. Today, her work at SickKids as an Infection Control Practitioner is shaped by her experiences as a parent at the hospital.
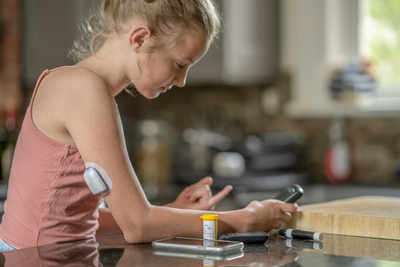
June 17, 2025
Clinical trial for teens shows improved health outcomes for type 1 diabetes
A new study in teenagers with type one diabetes shows promise in reducing chronic kidney disease and informing future precision care.